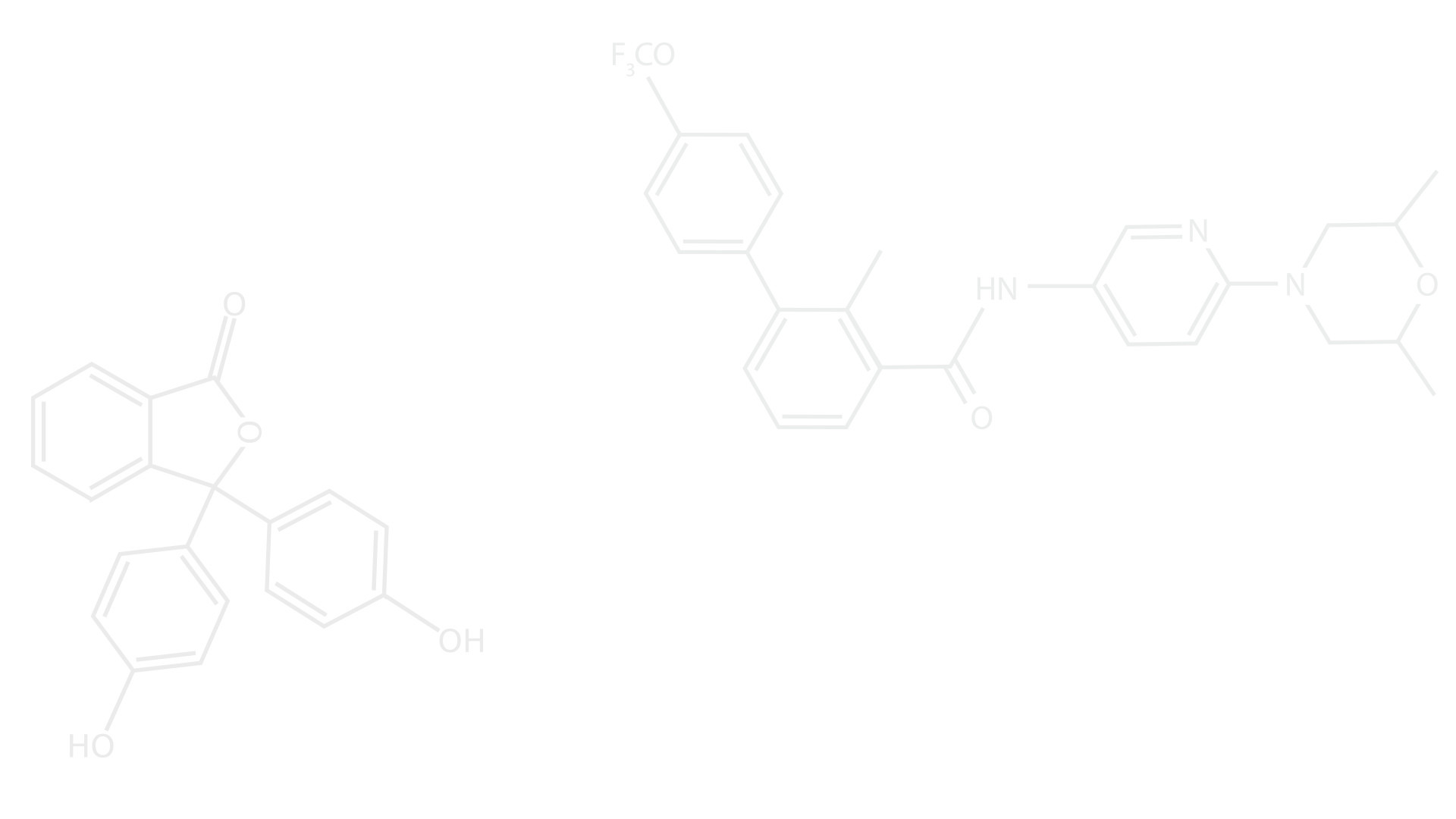
Research
Our laboratory seeks to understand how neuron-glia communication facilitates the formation, elimination and plasticity of synapses—the points of communication between neurons—during both healthy development and disease. A major goal is to elucidate the cellular and molecular mechanisms underlying activity-dependent synapse elimination during health and disease, with emphasis on the role of microglia and immune molecules in this process. Using the visual system as our primary model system, we employ a combination of live imaging, molecular, biochemical and neuroanatomical approaches.
IMMUNE CELLS IN THE HEALTHY AND DISEASED BRAIN
Microglia are brain parenchyma-resident macrophages that critically regulate neurodevelopment and whose dysregulation has been linked to the etiology of neurodevelopmental disorders and neurodegeneration. A major research focus of the lab is to understand how microglia interact with other cell types of the brain to regulate neurodevelopment and how dysregulation of these interactions contributes to neurological disease.
Furthermore, we wish to identify molecules that
instruct how microglia recognize and engulf
synapses, investigate the novel functions that
are performed by distinct microglia
subpopulations, explore contributions of other
immune populations and their interactions with
microglia to maintain health brain functionality
and respond to brain injury, and define how
microglia and other immune cells contribute to
neurodegenerative disorders. The synaptic
pruning functions of microglia are critical for
normal development and establishment of
neural circuits. Importantly, synapse loss and aberrant connectivity are common features of neuropsychiatric disorders, such as autism and schizophrenia, as well as neurodegenerative diseases, that exhibit both high levels of inflammation and microglial activation, such as Alzheimer’s disease and multiple sclerosis. The ability to ask detailed mechanistic questions about microglial engulfment of synaptic material in these contexts relies on the availability of robust and reproducible tools, which we are working to further develop.
MICROGLIA STATES AND FUNCTION
In addition to pruning synapses for proper brain wiring, microglia have been shown to perform other critical functions in the developing and healthy brain. These include promoting the survival of other brain cells, clearing unnecessary and damaging debris and ensuring that neurons communicate rapidly with each other. Our previous work has identified the presence of subpopulations of microglia that each express a unique combination of genes, which suggests that they contribute to distinct functions. For example, we identified a microglial subpopulation that is only detected during the first week of life in mice and is only found in structures called axon tracts. These Axon-Tract associated Microglia (ATM) express genes with unknown functions that have been linked to neurodevelopmental disorders such as autism. Moreover, axon tracts defects have been identified in patients with autism. Intriguingly, many of the genes expressed in ATM are also increased in microglia that are triggered in response to neurodegeneration. Another population called chemokine-secreting microglia express genes that are important for triggering an immune response. These microglia are low in number in the developing brain but increase with aging and in injury.
To dissect the contributions of these microglial subsets, we will use mouse models lacking key functional genes and investigate how loss of these genes alters microglial behavior, the behavior of the surrounding cells and the structural features of the brain where they reside. We will use live-imaging of labeled microglia from these subpopulations to specifically track and observe how they physically interact with their environment. We will also use cutting-edge sequencing tools to determine the mechanisms governing the precise window of emergence of microglial populations such as ATM. Understanding the specific functions and regulation of these microglial subpopulations to the brain will broaden our understanding of how microglia regulate normal neurodevelopment; in turn, these insights will inform our understanding of how their dysregulation leads to neuropsychiatric and neurodegenerative disease.
HOW ARE SYNAPSES ELIMINATED IN DISEASE?
Another major goal is to understand the signals that regulate the complement expression and function. We found that an astrocyte-derived factor (Factor X) up regulates C1q in developing retinal neurons during synapse elimination. Moreover, a dramatic up-regulation of complement occurs in glaucoma, Alzheimer’s, and other CNS diseases concurrently with the appearance of reactive astrocytes and microglia (Howell et al., JCI 2011) (Stephan et al., Annu. Rev. Neurosci. 2012).
Although synapse elimination is largely considered a developmental process, early synapse loss and dysfunction are becoming increasingly recognized as a hallmark of many CNS neurodegenerative diseases; however the factors that trigger synapse loss in the aged and diseased brain remain elusive.
We hypothesize that synapse loss in CNS neurodegenerative diseases is caused by a reactivation, in the mature brain, of similar developmental mechanisms of synapse elimination. Indeed, components of the complement cascade are profoundly up-regulated in Alzheimer’s Disease, glaucoma, and other brain diseases and are localized to synapses prior to signs of neuronal loss in animal models of neurodegenerative disease
In addition to our interest in CNS neurodegenerative diseases, we are probing the potential link between complement proteins and synapse loss in the pathogenesis of epilepsy and neurodevelopmental disorders.